New Models of Physics Instruction
Based on Physics Education Research: Part 2
by
Edward F. Redish
Department of Physics and Astronomy
University of Maryland
College Park MD 20742-4111
This paper appeared in Proceedings of the Deustchen Physikalischen Gesellschaft Jena Conference (1996).
It is displayed in two parts.
Go to part 1.
Some Research-Based Active-Engagement Instructional Methods
Over the past few years, a number of curricula have been developed in the USA that are based on the constructivist model of student thinking and learning and which have evolved using the research/curriculum reform/instruction cycle. I refer to these as active engagement classes. They all have in common a focus on what it is the students actually do and on what the effect of that activity is. A few examples of active engagement classes are:
Full Studio Models
- Physics by Inquiry (Lillian McDermott, et al., University of Washington)
- Workshop Physics (Priscilla Laws, Dickinson College)
- The Physics Studio (Jack Wilson, Rensselaer Polytechnic Institution)
Discovery Labs
- Tools for Scientific Thinking (R. Thornton, Tufts; D. Sokoloff, U. of Oregon)
- RealTime Physics (R. Thornton, Tufts; D. Sokoloff, U. of Oregon and P. Laws, Dickinson College)
Lecture Based Models
- Active Learning Physics System (Alan van Heuvelen, Ohio State University)
- Peer Instruction/ConcepTests (Eric Mazur, Harvard University)
- Interactive Demos (R. Thornton, Tufts; D. Sokoloff, U. of Oregon)
Recitation Based Models
- Cooperative Problem Solving (Ken and Pat Heller, University of Minnesota)
- Tutorials in Introductory Physics (Lillian McDermott, et al., University of Washington)
- Mathematical Tutorials (E. Redish et al., University of Maryland)
The traditional model of introductory university physics has a number of characteristics. As taught in the USA it has the following common features.
- It is content oriented.
- It has 3-4 hours of lecture and 1-0 hours of problem solving recitation per week.
- If there is a laboratory, it will be 2-3 hours and "cookbook" in nature; that is, students will go through a prescribed series of steps in order to demonstrate the truth of something taught in lecture or read in the book.
- The instructor is active during the class session while the students are passive during the class period (at least during lectures, and often during recitation).
- The instructor expects the student to undergo active learning activities outside of the class section, in reading, problem solving, etc.
The focus of the class is, in practice for most students, the lecture. The nature of this experience can be seen clearly in the structure of the classroom. A typical lecture room is illustrated schematically in Fig. 6.

Fig. 6: The structure of a typical lecture classroom.7
All students are turned to face the lecturer -- the focus of all attention. An active engagement class has somewhat different characteristics.
- The course is student oriented.
- What the students are actually doing in class is the focus of the course.
- Laboratories in this model are of the "discovery" type; that is, students are guided to observe phenomena and build for themselves the fundamental idea via observation.
- The course may include explicit training of reasoning.
- The student is expected to be intellectually active during the class.
I have grouped the active engagement classes I will discuss into four groups. In the full studio classes, the entire class time is taken up by periods in which the students are actively engaged with exploring the physics using some laboratory equipment. Only a small fraction of the period may be spent with a teacher lecturing to the students. These classes tend to be more expensive, both in terms of faculty time, space, and equipment required than the traditional lecture format. Other models of instruction have therefore been developed that replace one or more of the elements of the traditional structure by an active engagement activity. Laboratory-based models replace the traditional laboratory by a discovery type laboratory. Recitation-based models replace the recitation in which an instructors models problem solving for an hour by a mini-lab in which the students carry out guided discovery experiments and learn reasoning in groups guided by worksheets. Lecture-based models retain the timing and the lecture hall, but modify the activities carried out by the students during lecture.
Full Studio Models
Physics by Inquiry
One of the earlier prototypes of the full studio courses was Physics by Inquiry,8 developed by Lillian McDermott and her colleagues at the University of Washington over a period of nearly two decades. The course was developed for students studying to be teachers (pre-service teachers in the American terminology). The course is a full guided discovery laboratory. There is no lecture, only two laboratory periods of two hours each. During these periods, students work in pairs with simple equipment and are guided to reason through physical examples with simple apparatus and carefully prepared worksheets. A sample apparatus for the unit on light is shown in Fig. 7.
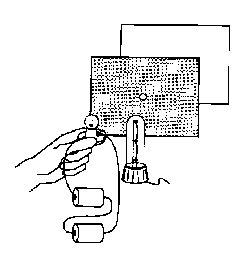
Fig. 7: A simple apparatus from Physics by Inquiry.
The worksheets are based on research in student understanding and try to put the students in situations where their confusions will be elicited in their predictions of how a system will behave. When the system fails to behave as the student predicts, a cognitive conflict results. Trained facilitators (approximately one for every 10-15 students) help students to find their own path to understanding by guiding them with carefully chosen questions.
The structure of the classroom (see Fig. 8) illustrates the fundamental difference between this class and the traditional lecture. The focus of the students' attention is clearly the equipment and the interaction of the group, not the instructor.
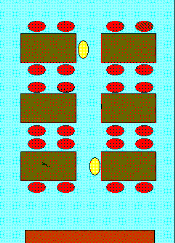
Fig. 8: The structure of a typical inquiry-based course classroom.
Workshop Physics/Physics Studio
Among the groups that have developed inquiry style classes for the calculus-based university physics course, two stand out. Both the Workshop Physics9 class developed at Dickinson College by Priscilla Laws, and the Physics Studio10 developed at Rensselaer Polytechnic Institute by Jack Wilson, make strong use of computer equipment to give the student a more quantitative view of the world. What it is the students actually do in this class is hinted at by the structure of the classroom, shown in Fig. 9.

Fig. 9: A typical workshop or studio classroom layout.
The students function in groups as in the inquiry-style classroom, but each pair of students works with a computer connected to an analog to digital conversion device such as the Universal Lab Interface box (ULI).11 A variety of probes can be connected to the box, including position or angle detectors, force probes, pressure and voltage sensors, etc. The computer stations also contain calculational and modeling tools such as a spreadsheet, programming language, and symbolic manipulator. These classes are also held in two hour periods in which most of the student time is spent with apparatus making observations and building mathematical models of their results. The classroom contains a central area for common demonstrations and many class periods may include brief lecture segments or whole-class discussions. These classes use worksheets (in Workshop Physics) or on-screen lessons (in the Physics Studio) to help guide the student through the process of carrying out, making sense of, and modeling their experiments. In many cases, the experiments will be enabled by cleverly designed apparatus that, in conjunction with the ULI data acquisition probes, provides the student with a simple and direct quantitative view of what otherwise might be an obscure and confusing long chain of inferences. In Fig. 10 we show a "chaos machine" from Workshop Physics.12

Fig. 10: A "chaos machine"
The device is a van der Pol oscillator -- a wheel containing an edge-weight that produces a non-linear restoring force. It is driven by a controllable motor attached to a spring. The wheel is mounted on a "smart pulley" that connects to the ULI and measures the angle of displacement. Phase plots can be produced on the computer screen in real time and the students can see how they change in response to a change of the system's parameters. The transition to chaos can be seen, both in what it means for the real system and for how it appears in the phase space plot. Screen captures for two different sets of parameters are shown below illustrating bifurcation and the approach to chaos.

Fig. 11: Data from the chaos machine taken with two different sets of system parameters.13
Discovery Labs
The lab is the single item in a traditional physics course where the student is expected to be actively engaged during the class period. Unfortunately, in many cases the laboratory has turned into a place to either "demonstrate the truth of something taught in lecture" or a place to "produce a good result". The focus in both of these cases is on the content and not on what might be valuable for a student to learn from the activity. In the USA, "cookbook" laboratories -- ones in which highly explicit instructions are given and the student doesn't have to think -- are common. They are unpopular with students and tend to produce little learning. A number of interesting "guided discovery labs" have been developed in the past few years that appear to be more effective.
Tools for Scientific Thinking
Ron Thornton at Tufts University and David Sokoloff at Oregon State have developed Tools for Scientific Thinking14 -- a series of guided discovery laboratories in the areas of mechanics and thermodynamics. These units focus on concept building and overcoming those student misconceptions and difficulties that researchers have found to be common. These laboratories rely on computer-data-acquisition equipment similar to that of Workshop Physics but are created as modules which can be used in a more traditional laboratory format. They make extensive use of cognitive conflict and peer interaction. Thornton and Sokoloff have done extensive research to demonstrate the effectiveness of this approach.15 These materials are appropriate for the high school and introductory university level and focus on a conceptual rather than quantitative approach.
RealTime Physics
Thornton, Sokoloff, and Laws have recently combined to develop a new series of mechanics laboratories that can be used in a traditional structure.16 These are similar in spirit to both Tools for Scientific Thinking and Workshop Physics. Heavy use is made of computer assisted data acquisition and the results of research on student difficulties. A more quantitative approach appropriate for calculus-based physics is developed.
Lecture Based Models
A number of interactive-engagement classes have been developed that work within the lecture format.
Peer Instruction ConcepTests
Eric Mazur at Harvard University17 has modified his lectures by including three to four "concept tests" in each hour of lecture. After a 10-15 minute lecture segment, he presents a challenging multiple choice question to the class. This question is concept oriented and the distractors are based on the most common student difficulties as shown by research. Students answer the questions at their seats using a device that collects and displays the collective response on a projection screen, such as ClassTalk. As a result of the careful choice of question and distractors, the class usually is divided as to what they believe is the correct answer. Mazur then instructs the students to discuss the problem with their neighbor for 2-3 minutes. At the end of this period, the students answer the question again. Usually the discussion has produced a substantial improvement. If not, Mazur presents additional material.
The combination of research-based concept tests with peer interaction makes these lectures into an active-engagement environment for the student. The display of the distribution of class results may play an important role.
Interactive Demos
One recent development that may prove both effective and efficient is a series of interactive lecture demonstrations for mechanics by Thornton and Sokoloff. They have adapted their successful microcomputer-based laboratory curricula and used the results of their research into student learning to create a series of demonstrations that focus on the issues that are fundamental to student understanding of mechanics. These demonstrations are delivered to a large lecture by trained demonstrators for a few lecture periods during a semester. In order to get the students actively engaged, they have each student fill out a worksheet during the demonstration. The students are called on to make (and write down) their predictions and are led to discuss the results for a few minutes with their neighbors as in the Mazur method. Preliminary results show very strong improvement compared to normal non-interactive lecture classes.
ALPS
Alan van Heuvelen at Ohio State18 has developed a series of worksheets for use in a large lecture format. Small bits of lecture are alternated with individual student activities and peer discussion, as in Mazur's model. This is similar in spirit to the other two lecture models discussed above but does not rely on heavy (and expensive) doses of technology.
Recitation-Based Models
Two models have obtained significant improvement in building students' conceptual understanding with a limited amount of modification of the traditional model. They only introduce interactive engagement activities in place of the recitation section, one hour per week.
Cooperative-Problem Solving
Pat and Ken Heller at the University of Minnesota19 and their collaborators have developed a group-learning problem-solving environment in which students work together in recitation on problems they have not previously seen. These problems are "context rich", that is, they involve realistic situations, may contain incomplete data, and may require the students to pose a part of the problem themselves. The problems are intended to be too difficult for any individual student to solve. Groups are formed to include students of varying ability and students may be assigned specific (and rotating) roles to play in each group.
Recently, the Hellers have extended their method to include the laboratory and have modified some lectures to be more interactive. The combined results seem to be highly effective.20
Tutorials in Introductory Physics
McDermott and her group have developed a method for introducing inquiry type sessions into recitations.21 The traditional "the instructor models problem-solving while the students watch passively" is replaced by group learning activity with carefully designed research-based worksheets. These worksheets emphasize concept building, qualitative reasoning, and make use of cognitive conflict with trained facilitators to assist in helping students resolve their own confusions.
At the University of Maryland we have developed a series of tutorials in this framework that uses the data acquisition tools of the studio classes and focuses on the use of mathematical concepts in physics. In tutorials, specific student conceptual difficulties are targeted.
The crucial element in each of these courses is that they have been developed as a result of detailed attention to student learning as well as course content. Some are associated with specific and detailed physics education research.
A Sample Evaluation
Many of these methods have been demonstrated to be effective in improving student understanding of fundamental physics concepts. Although there is insufficient space here to discuss all the evaluation that has been done, some of the references given that describe the methods in detail also include discussions of their evaluation. I will report here on one measurement we have made testing the effectiveness of tutorials.22 We use the Force Concept Inventory23 (FCI) which is a 29 question multiple choice test designed to probe the students' conceptual understanding of force, dynamics, and some kinematics. It was developed on the basis of explicit and detailed physics education research. The diabolical aspect of the test is that the "distractors" (wrong answers) are the most commonly found misconceptions. Students who are confused or not confident of their understanding of Newtonian mechanics are often tempted to give one of these wrong answer.
A study24 of the FCI given at many universities and high schools in the USA shows a systematic behavior. Courses of a similar structure (as judged on what it is the students actually do in the course) show similar "fractions of the possible gain" when the tests are given before and after instruction, despite wide initial differences between pre-test scores. The figure of merit that we use is therefore:
h = fraction of the possible gain
h = (post test class average - pre-test class average)/(100 - pre-test class average)

Fig. 12: Figure of merit histogram. h = fraction of the possible gain obtained on the FCI for tutorial (green bars) and recitation (red bars) classes.13
We tested tutorials in classes of engineering physics at the University of Maryland from 1993-95. The FCI was given as pre and post-tests in ten lecture sections of first semester calculus-based physics. Five sections used tutorials, five did not. Six different professors participated in the study. The results are shown in Fig. 12. Every one of the tutorial classes scored better than every one of the non-tutorial classes. We also obtained more detailed results on the results of individual MBL tutorials on the concept of instantaneous velocity and Newton's third law.
Students who had tutorials -- even only a single hour -- performed significantly better on our evaluation of their conceptual understanding of those specific topics than those students who had recitation.
Conclusion
The growing demand that we teach physics more effectively has led to an explosion of development and innovation. Many promising new courses will fail, as many promising educational ideas have failed in the past. Only by building a knowledge base strongly supported by evidence can we escape the draw of faddism / fashion and begin to make shared, cumulative progress.
We as physics teachers must begin to make for ourselves the "gestalt shift" to see our physics classroom in a new way. The student takes on a more "visible" role in what is happening in the classroom. The content does not diminish in importance, but the student's relation with it takes on a new and primary significance.
Acknowledgments
I would like to thank Prof. Dr. H. J. Schlichting, Prof. Dr. K.-H. Lotze, Prof. Dr. Gunther Kurze, and the other officers of the DPG for inviting me to the Jena Conference. I would like to thank Richard Steinberg for a careful reading of the manuscript. This work is supported in part by grants from the US National Science Foundation.
7 This figure courtesy of Jack M. Wilson, Rensselaer Polytechnic Institute.
8 Lillian C. McDermott et al., Physics by Inquiry (John Wiley and Sons, NY, 1996).
9 Priscilla Laws, "Calculus-based physics without lectures," Phys. Today 44:12 (1991) 24-31; Priscilla Laws, Workshop Physics (Vernier Software, Portland OR, 1995).
10 Jack M. Wilson, "The CUPLE Physics Studio", The Physics Teacher 32 (1994) 518-529.
11 Vernier Software, Portland OR.
12 The chaos machine is currently available from Pasco.
13 Data taken by Bao Lei, University of Maryland.
14 David R. Sokoloff and Ronald K. Thornton, Tools for Scientific Thinking (Vernier Software, Portland OR, 1992 and 1993).
15 Ronald K. Thornton, "Conceptual Dynamics: Changing Student Views of Force and Motion," in C. Tarsitani, C. Bernardini and M.Vincentini, (Eds.) Thinking Physics for Teaching (Plenum, 1995).
16 Priscilla Laws, David R. Sokoloff and Ronald K. Thornton, RealTime Physics (Vernier Software, Portland OR, 1995).
17 Eric Mazur, Peer Interaction, A User's Manual (Prentice Hall, 1996).
1
18 Alan Van Heuvelen, ALPS: Mechanics (Vol. 1), Electricity and Magnetism (Vol. 2) (Hayden-McNeil Publishing, Westland MI, 1994).
19 Patricia Heller, Ronald Keith, and Scott Anderson, "Teaching problem solving through cooperative grouping. Part 1: Group versus individual problem solving," Am. J. Phys. 60:7 (1992) 627-636; Patricia Heller, and Mark Hollabaugh, "Teaching problem solving through cooperative grouping. Part 2: Designing problems and structuring groups," Am. J. Phys. 60:7 (1992) 637-644.
20 Ken Heller, private communication, January 1996.
21 Lillian C. McDermott, Peter S. Shaffer, and Mark D. Somers, "Research as a guide for teaching introductory mechanics: An illustration in the context of the Atwoods's machine," Am. J. Phys. 62 (1994) 46-55.
22 Edward F. Redish, Jeffery M. Saul, and Richard N. Steinberg, "On the effectiveness of active-engagement microcomputer-based laboratories", University of Maryland preprint, to be published.
23 David Hestenes, Malcolm. Wells, and Gregg Swackhammer, "Force Concept Inventory," The Physics Teacher 30:3 (1992) 141-158.
24 Richard Hake, "A five-thousand-student survey of mechanics test data for introductory physics courses", Indiana University preprint, to be published.
Go to part 1.
This page prepared 9. June 1996 by
Edward F. Redish
Department of Physics
University of Maryland
College Park, MD 20742
Phone: (301) 405-6120
redish@physics.umd.edu